- Neotipo
- Este blog será el testigo del proceso creativo y, a la par, subiré los avances narrativos en entregas.
viernes, 30 de enero de 2015
martes, 27 de enero de 2015
domingo, 25 de enero de 2015
sábado, 24 de enero de 2015
miércoles, 21 de enero de 2015
martes, 20 de enero de 2015
The universe according to Nietzsche: Modern cosmology and the theory of eternal recurrence
PAUL STEINHARDT

Excerpted from "The Universe: Leading Scientists Explore the Origin, Mysteries, and Future of the Cosmos." It originally appeared as a speech given by Steinhardt at an event in 2002.
If you were to ask most cosmologists to give a summary of where we stand right now in the field, they would tell you that we live in a very special period in human history where, thanks to a whole host of advances in technology, we can suddenly view the very distant and very early universe in ways we haven’t been able to do ever before. For example, we can get a snapshot of what the universe looked like in its infancy, when the first atoms were forming. We can get a snapshot of what the universe looked like in its adolescence, when the first stars and galaxies were forming. And we are now getting a full detail, three-dimensional image of what the local universe looks like today. When you put together this different information, which we’re getting for the first time in human history, you obtain a very tight series of constraints on any model of cosmic evolution.
If you go back to the different theories of cosmic evolution in the early 1990s, the data we’ve gathered in the last decade has eliminated all of them save one, a model that you might think of today as the consensus model. This model involves a combination of the Big Bang model as developed in the 1920s, ’30s, and ’40s; the inflationary theory, which Alan Guth proposed in the 1980s; and a recent amendment that I will discuss shortly. This consensus theory matches the observations we have of the universe today in exquisite detail. For this reason, many cosmologists conclude that we have finally determined the basic cosmic history of the universe.
But I have a rather different point of view, a view that has been stimulated by two events. The first is the recent amendment to which I referred earlier. I want to argue that the recent amendment is not simply an amendment but a real shock to our whole notion of time and cosmic history. And secondly, in the last year I’ve been involved in the development of an alternative theory that turns the cosmic history topsy-turvy: All the events that created the important features of our universe occur in a different order, by different physics, at different times, over different time scales. And yet this model seems capable of reproducing all the successful predictions of the consensus picture with the same exquisite detail.
The key difference between this picture and the consensus picture comes down to the nature of time. The standard model, or consensus model, assumes that time has a beginning that we normally refer to as the Big Bang. According to that model, for reasons we don’t quite understand, the universe sprang from nothingness into somethingness, full of matter and energy, and has been expanding and cooling for the past 15 billion years. In the alternative model, the universe is endless. Time is endless, in the sense that it goes on forever in the past and forever in the future, and in some sense space is endless. Indeed, our three spatial dimensions remain infinite throughout the evolution of the universe.
More specifically, this model proposes a universe in which the evolution of the universe is cyclic. That is to say, the universe goes through periods of evolution from hot to cold, from dense to under-dense, from hot radiation to the structure we see today, and eventually to an empty universe. Then, a sequence of events occurs that cause the cycle to begin again. The empty universe is reinjected with energy, creating a new period of expansion and cooling. This process repeats periodically forever. What we’re witnessing now is simply the latest cycle.
The notion of a cyclic universe is not new. People have considered this idea as far back as recorded history. The ancient Hindus, for example, had a very elaborate and detailed cosmology based on a cyclic universe. They predicted the duration of each cycle to be 8.64 billion years—a prediction with three-digit accuracy. This is very impressive, especially since they had no quantum mechanics and no string theory! It disagrees with the number I’m going suggest, which is trillions of years rather than billions.
The cyclic notion has also been a recurrent theme in Western thought. Edgar Allan Poe and Friedrich Nietzsche, for example, each had cyclic models of the universe, and in the early days of relativistic cosmology Albert Einstein, Alexander Friedmann, Georges Lemaître, and Richard Tolman were interested in the cyclic idea. I think it’s clear why so many have found the cyclic idea to be appealing: If you have a universe with a beginning, you have the challenge of explaining why it began and the conditions under which it began. If you have a universe that’s cyclic, it’s eternal, so you don’t have to explain the beginning.
During the attempts to try to bring cyclic ideas into modern cosmology, it was discovered in the 1920s and ’30s that there are various technical problems. The idea at that time was a cycle in which our three-dimensional universe goes through periods of expansion beginning from the Big Bang and then reversal to contraction and a Big Crunch. The universe bounces, and expansion begins again. One problem is that every time the universe contracts to a crunch, the density and temperature of the universe rises to an infinite value, and it is not clear if the usual laws of physics can be applied.
Second, every cycle of expansion and contraction creates entropy through natural thermodynamic processes, which adds to the entropy from earlier cycles. So at the beginning of a new cycle, there is higher entropy density than the cycle before. It turns out that the duration of a cycle is sensitive to the entropy density. If the entropy increases, the duration of the cycle increases as well. So, going forward in time, each cycle becomes longer than the one before. The problem is that, extrapolating back in time, the cycles become shorter until, after a finite time, they shrink to zero duration. The problem of avoiding a beginning has not been solved; it has simply been pushed back a finite number of cycles. If we’re going to reintroduce the idea of a truly cyclic universe, these two problems must be overcome. The cyclic model I will describe uses new ideas to do just that.
To appreciate why an alternative model is worth pursuing, it’s important to get a more detailed impression of what the consensus picture is like. Certainly some aspects are appealing. But what I want to argue is that, overall, the consensus model is not so simple. In particular, recent observations have forced us to amend the consensus model and make it more complicated. So, let me begin with an overview of the consensus model.
The consensus theory begins with the Big Bang: The universe has a beginning. It’s a standard assumption that people have made over the last fifty years, but it’s not something we can prove at present from any fundamental laws of physics. Furthermore, you have to assume that the universe began with an energy density less than the critical value. Otherwise, the universe would stop expanding and recollapse before the next stage of evolution, the inflationary epoch. In addition, to reach this inflationary stage, there must be some sort of energy to drive the inflation. Typically this is assumed to be due to an inflation field. You have to assume that in those patches of the universe that began at less than the critical density, a significant fraction of the energy is stored in inflation energy so that it can eventually overtake the universe and start the period of accelerated expansion. All of these are reasonable assumption, but assumptions nevertheless. It’s important to take into account these assumptions and ingredients, because they’re helpful in comparing the consensus model to the challenger.
Assuming these conditions are met, the inflation energy overtakes the matter and radiation after a few instants. The inflationary epoch commences, and the expansion of the universe accelerates at a furious pace. The inflation does a number of miraculous things: It makes the universe homogeneous, it makes the universe flat, and it leaves behind certain inhomogeneities, which are supposed to be the seeds for the formation of galaxies. Now the universe is prepared to enter the next stage of evolution with the right conditions. According to the inflationary model, the inflation energy decays into a hot gas of matter and radiation. After a second or so, there form the first light nuclei. After a few tens of thousands of years, the slowly moving matter dominates the universe. It’s during these stages that the first atoms form, the universe becomes transparent, and the structure in the universe begins to form—the first stars and galaxies. Up to this point, the story is relatively simple.
But there is the recent discovery that we’ve entered a new stage in the evolution of the universe. After the stars and galaxies have formed, something strange has happened to cause the expansion of the universe to speed up again. During the 15 billion years when matter and radiation dominated the universe and structure was forming, the expansion of the universe was slowing down, because the matter and radiation within it is gravitationally self-attractive and resists the expansion of the universe. Until very recently, it had been presumed that matter would continue to be the dominant form of energy in the universe and this deceleration would continue forever.
But we’ve discovered instead, due to recent observations, that the expansion of the universe is speeding up. This means that most of the energy of the universe is neither matter nor radiation. Rather, another form of energy has overtaken the matter and radiation. For lack of a better term, this new energy form is called dark energy. Dark energy, unlike the matter and radiation we’re familiar with, is gravitationally self-repulsive. That’s why it causes the expansion to speed up rather than slow down. In Newton’s theory of gravity, all mass is gravitationally attractive, but Einstein’s theory allows the possibility of forms of energy that are gravitationally self-repulsive.
I don’t think either the physics or cosmology communities, or even the general public, have fully absorbed the full implications of this discovery. This is a revolution in the grand historic sense—in the Copernican sense. In fact, if you think about Copernicus—from whom we derive the word “revolution”—his importance was that he changed our notion of space and of our position in the universe. By showing that the Earth revolves around the sun, he triggered a chain of ideas that led us to the notion that we live in no particular place in the universe; there’s nothing special about where we are. Now we’ve discovered something very strange about the nature of time: that we may live in no special place, but we do live at a special time, a time of recent transition from deceleration to acceleration; from one in which matter and radiation dominate the universe to one in which they are rapidly becoming insignificant components; from one in which structure is forming in ever larger scales to one in which now, because of this accelerated expansion, structure formation stops. We are in the midst of the transition between these two stages of evolution. And just as Copernicus’ proposal that the Earth is no longer the center of the universe led to a chain of ideas that changed our whole outlook on the structure of the solar system and eventually to the structure of the universe, it shouldn’t be too surprising that perhaps this new discovery of cosmic acceleration could lead to a whole change in our view of cosmic history. That’s a big part of the motivation for thinking about our alternative proposal.
With these thoughts about the consensus model in mind, let me turn to the cyclic proposal. Since it’s cyclic, I’m allowed to begin the discussion of the cycle at any point I choose. To make the discussion parallel, I’ll begin at a point analogous to the Big Bang; I’ll call it the Bang. This is a point in the cycle where the universe reaches its highest temperature and density. In this scenario, though, unlike the Big Bang model, the temperature and density don’t diverge. There is a maximal, finite temperature. It’s a very high temperature, around 1020 degrees Kelvin—hot enough to evaporate atoms and nuclei into their fundamental constituents—but it’s not infinite. In fact, it’s well below the so-called Planck energy scale, where quantum gravity effects dominate. The theory begins with a bang and then proceeds directly to a phase dominated by radiation. In this scenario you do not have the inflation one has in the standard scenario. You still have to explain why the universe is flat, you still have to explain why the universe is homogeneous, and you still have to explain where the fluctuations came from that led to the formation of galaxies, but that’s not going to be explained by an early stage of inflation. It’s going to be explained by yet a different stage in the cyclic universe, which I’ll get to.
In this new model, you go directly to a radiation-dominated universe and form the usual nuclear abundances; then go directly to a matter-dominated universe in which the atoms and galaxies and larger-scale structure form; and then proceed to a phase of the universe dominated by dark energy. In the standard case, the dark energy comes as a surprise, since it’s something you have to add into the theory to make it consistent with what we observe. In the cyclic model, the dark energy moves to center stage as the key ingredient that is going to drive the universe, and in fact drives the universe, into the cyclic evolution. The first thing the dark energy does when it dominates the universe is what we observe today: It causes the expansion of the universe to begin to accelerate. Why is that important? Although this acceleration rate is 100 orders of magnitude smaller than the acceleration that one gets in inflation, if you give the universe enough time it actually accomplishes the same feat that inflation does. Over time, it thins out the distribution of matter and radiation in the universe, making the universe more and more homogeneous and isotropic—in fact, making it perfectly so—driving it into what is essentially a vacuum state.
Seth Lloyd said there were 1080 or 1090 bits inside the horizon, but if you were to look around the universe in a trillion years, you would find on average no bits inside your horizon, or less than one bit inside your horizon. In fact, when you count these bits, it’s important to realize that now that the universe is accelerating, our computer is actually losing bits from inside our horizon. This is something that we observe.
At the same time that the universe is made homogeneous and isotropic, it is also being made flat. If the universe had any warp or curvature to it, or if you think about the universe stretching over this long period of time, although it’s a slow process it makes the space extremely flat. If it continued forever, of course, that would be the end of the story. But in this scenario, just like inflation, the dark energy survives only for a finite period and triggers a series of events that eventually lead to a transformation of energy from gravity into new energy and radiation that will then start a new period of expansion of the universe. From a local observer’s point of view, it looks like the universe goes through exact cycles; that is to say, it looks like the universe empties out each round and a new matter and radiation is created, leading to a new period of expansion. In this sense it’s a cyclic universe. If you were a global observer and could see the entire universe, you’d discover that our three dimensions are forever infinite in this story. What’s happened is that at each stage when we create matter and radiation, it gets thinned out. It’s out there somewhere, but it’s getting thinned out. Locally, it looks like the universe is cyclic, but globally the universe has a steady evolution, a well-defined era in which, over time and throughout our three dimensions, entropy increases from cycle to cycle.
Exactly how this works in detail can be described in various ways. I will choose to present a very nice geometrical picture that’s motivated by superstring theory. We use only a few basic elements from superstring theory, so you don’t really have to know anything about superstring theory to understand what I’m going to talk about, except to understand that some of the strange things I’m going to introduce I am not introducing for the first time. They’re already sitting there in superstring theory waiting to be put to good purpose.
One of the ideas in superstring theory is that there are extra dimensions; it’s an essential element to that theory, which is necessary to make it mathematically consistent. In one particular formulation of that theory, the universe has a total of eleven dimensions. Six of them are curled up into a little ball so tiny that, for my purposes, I’m just going to pretend they’re not there. However, there are three spatial dimensions, one time dimension, and one additional dimension that I do want to consider. In this picture, our three dimensions with which we’re familiar and through which we move lie along a hypersurface, or membrane. This membrane is a boundary of the extra dimension. There is another boundary, or membrane, on the other side. In between, there’s an extra dimension that, if you like, only exists over a certain interval. It’s like we are one end of a sandwich, in between which there is a so-called bulk volume of space. These surfaces are referred to as orbifolds or branes—the latter referring to the word “membrane.” The branes have physical properties. They have energy and momentum, and when you excite them you can produce things like quarks and electrons. We are composed of the quarks and electrons on one of these branes. And, since quarks and leptons can only move along branes, we are restricted to moving along and seeing only the three dimensions of our brane. We cannot see directly the bulk or any matter on the other brane.
In the cyclic universe, at regular intervals of trillions of years, these two branes smash together. This creates all kinds of excitations—particles and radiation. The collision thereby heats up the branes, and then they bounce apart again. The branes are attracted to each other through a force that acts just like a spring, causing the branes to come together at regular intervals. To describe it more completely, what’s happening is that the universe goes through two kinds of stages of motion. When the universe has matter and radiation in it, or when the branes are far enough apart, the main motion is the branes stretching, or, equivalently, our three dimensions expanding. During this period, the branes more or less remain a fixed distance apart. That’s what’s been happening, for example, in the last 15 billion years. During these stages, our three dimensions are stretching just as they normally would. At a microscopic distance away, there is another brane sitting and expanding, but since we can’t touch, feel, or see across the bulk, we can’t sense it directly. If there is a clump of matter over there, we can feel the gravitational effect, but we can’t see any light or anything else it emits, because anything it emits is going to move along that brane. We only see things that move along our own brane.
Next, the energy associated with the force between these branes takes over the universe. From our vantage point on one of the branes, this acts just like the dark energy we observe today. It causes the branes to accelerate in their stretching, to the point where all the matter and radiation produced since the last collision is spread out and the branes become essentially smooth, flat, empty surfaces. If you like, you can think of them as being wrinkled and full of matter up to this point, and then stretching by a fantastic amount over the next trillion years. The stretching causes the mass and energy on the brane to thin out and the wrinkles to be smoothed out. After trillions of years, the branes are, for all intents and purposes, smooth, flat, parallel, and empty.
Then the force between these two branes slowly brings the branes together. As it brings them together, the force grows stronger and the branes speed toward one another. When they collide, there’s a walloping impact—enough to create a high density of matter and radiation with a very high, albeit finite, temperature. The two branes go flying apart, more or less back to where they are, and then the new matter and radiation, through the action of gravity, causes the branes to begin a new period of stretching.
In this picture, it’s clear that the universe is going through periods of expansion and a funny kind of contraction. Where the two branes come together, it’s not a contraction of our dimensions but a contraction of the extra dimension. Before the contraction, all matter and radiation has been spread out, but, unlike the old cyclic models of the 1920s and ’30s, it doesn’t come back together again during the contraction, because our three dimensions—that is, the branes—remain stretched out. Only the extra dimension contracts. This process repeats itself cycle after cycle.
If you compare the cyclic model to the consensus picture, two of the functions of inflation—namely, flattening and homogenizing the universe—are accomplished by the period of accelerated expansion that we’ve now just begun. Of course, I really mean the analogous expansion that occurred one cycle ago, before the most recent Bang. The third function of inflation—producing fluctuations in the density—occurs as these two branes come together. As they approach, quantum fluctuations cause the branes to begin to wrinkle. And because they’re wrinkled, they don’t collide everywhere at the same time. Rather, some regions collide a bit earlier than others. This means that some regions reheat to a finite temperature and begin to cool a little bit before other regions. When the branes come apart again, the temperature of the universe is not perfectly homogeneous but has spatial variations left over from the quantum wrinkles.
Remarkably, although the physical processes are completely different and the time scale is completely different—this is taking billions of years, instead of 10-30 seconds—it turns out that the spectrum of fluctuations you get in the distribution of energy and temperature is essentially the same as what you get in inflation. Hence, the cyclic model is also in exquisite agreement with all of the measurements of the temperature and mass distribution of the universe that we have today.
Because the physics in these two models is quite different, there is an important distinction in what we would observe if one or the other were actually true—although this effect has not been detected yet. In inflation when you create fluctuations, you don’t just create fluctuations in energy and temperature but you also create fluctuations in spacetime itself, so-called gravitational waves. That’s a feature we hope to look for in experiments in the coming decades as a verification of the consensus model. In our model, you don’t get those gravitational waves. The essential difference is that inflationary fluctuations are created in a hyperrapid, violent process that is strong enough to create gravitational waves, whereas cyclic fluctuations are created in an ultraslow, gentle process that is too weak to produce gravitational waves. That’s an example where the two models give an observational prediction that is dramatically different. It’s just difficult to observe at the present time.
What’s fascinating at the moment is that we have two paradigms now available to us. On the one hand, they are poles apart in terms of what they tell us about the nature of time, about our cosmic history, about the order in which events occur, and about the time scale on which they occur. On the other hand, they are remarkably similar in terms of what they predict about the universe today. Ultimately what will decide between the two is a combination of observations—for example, the search for cosmic gravitational waves—and theory, because a key aspect to this scenario entails assumptions about what happens at the collision between branes that might be checked or refuted in superstring theory. In the meantime, for the next few years, we can all have great fun speculating about the implications of each of these ideas and how we can best distinguish between them.
Paul Steinhardt is a theoretical physicist, an Albert Einstein Professor of Science at Princeton University and coauthor (with Neil Turok) of “Endless Universe: Beyond the Big Bang.” This piece originally appeared as a speech by Steinhardt at an event in 2002. It has been excerpted here as it appears in “The Universe: Leading Scientists Explore the Origin, Mysteries, and Future of the Cosmos.” Copyright © 2014 by Edge Foundation Inc. Published by Harper Perennial
fuente: http://www.salon.com/2014/07/13/the_universe_according_to_nietzsche_modern_cosmology_and_the_theory_of_eternal_recurrence/
Piensa...
Piensa en todo lo que ha sucedido aquí, en esta tierra. En toda la sangre
ardiente y violenta y fuerte que ha perseguido la vida, el placer. También pasaron pesadumbre y sufrimiento, naturalmente, pero en cualquier caso sacando siempre algo de todo ello, mucho, porque en última instancia uno no tiene por qué seguir soportando aquello que considera sufrimiento, pues siempre puede poner fin a tales situaciones. E incluso el sufrimiento y la pesadumbre son mejores que nada; no hay nada peor que no estar vivo. Pero uno no puede vivir eternamente, uno siempre consume la vida antes de agotar todas las posibilidades de vivir. Y todo ello debe estar en alguna parte.
William Faulkner. Desciende, Moisés
ardiente y violenta y fuerte que ha perseguido la vida, el placer. También pasaron pesadumbre y sufrimiento, naturalmente, pero en cualquier caso sacando siempre algo de todo ello, mucho, porque en última instancia uno no tiene por qué seguir soportando aquello que considera sufrimiento, pues siempre puede poner fin a tales situaciones. E incluso el sufrimiento y la pesadumbre son mejores que nada; no hay nada peor que no estar vivo. Pero uno no puede vivir eternamente, uno siempre consume la vida antes de agotar todas las posibilidades de vivir. Y todo ello debe estar en alguna parte.
William Faulkner. Desciende, Moisés
La ciencia (y las explicaciones TED) detrá de Interestellar
Hace mucho no me conmocionaba, visual y sonora, una película como Interestellar.Así que, para todos los amantes del espacio y la física, les dejo estos videos TED que pueden ayudar a entender el trasfondo de la película de Nolan
The science of Interstellar: 5 TED-Ed Lessons to help you understand the film
- 13 November, 2014 //
- Education, General, List, Science //
- Posted By Alex Rosenthal
- 94 Comments
Thanks to physicist Kip Thorne’s influence, the latest space-travel film Interstellar features some remarkably scientifically accurate depictions of topics that have previously been mangled by the Hollywood treatment. Here, we wrangled together five TED-Ed Lessons that can serve as a (spoiler-filled) crash course on some of the trickier science in the film.
Time Dilation
Why do 7 years pass on Earth for every hour spent on the water planet orbiting Gargantua? How does Murph wind up older than her father? Why does Paul Rudd never seem to age? (OK, that last one has nothing to do with Interstellar, but we have our suspicions that they’re related…)
5-dimensional Beings
What does it actually mean for a being to be 5 dimensional? What is the tesseract that Coop falls into inside the Black Hole’s event horizon?
*Note: This lesson references 4 dimensional life forms, while Interstellar talks about 5 dimensional beings. The reason for the difference is that this lesson is just looking at geometric dimensions, whereas Insterstellar is counting 4 spatial dimensions plus 1 time dimension.
*Note: This lesson references 4 dimensional life forms, while Interstellar talks about 5 dimensional beings. The reason for the difference is that this lesson is just looking at geometric dimensions, whereas Insterstellar is counting 4 spatial dimensions plus 1 time dimension.
Black Holes and Gravity
Why is there a secret locked inside Gargantua? How are gravity and spacetime related? Why does Sandra Bullock hate space so much? (OK, OK, different movie again, sorry!)
Tidal Waves
We suspect that the colossal waves on the water planet have something to do with tidal forces caused by Gargantua’s gravitational pull, similar to how the moon causes the Earth’s tides. The film isn’t entirely clear on that point, but for an explanation of tsunamis on Earth, look no further.
Newton’s Third Law
It isn’t often that a protagonist will cite a foundational law of physics when he heroically sacrifices himself. But when he or she does, it makes us feel all warm and fuzzy inside. This video will help you brush up on Newton’s Laws for the next time you need to justify an epic life decision.
Traducir
Fuente: http://www.space.com/26964-nasa-mega-rocket-2018-sls-test-launch.html?adbid=10152472849486466&adbpl=fb&adbpr=17610706465&cmpid=514630_20141127_31114266
NASA new mega-rocket, a towering booster designed for deep space missions, will be ready for its first test flight no later than November 2018, space agency officials announced Wednesday (Aug. 27).
It's possible that the Space Launch System rocket test flight could launch as early as December 2017, but NASA officials have committed to having the rocket ready for flight be the end of 2018 to be safe. That extra wiggle room should let the space agency cope with scheduling and funding issues as they crop up in the future, NASA officials said in a teleconference with reporters.
The SLS will be the largest rocket ever constructed and it is designed to send humans deeper into space than ever before. The huge launcher — which will stand at 400-feet-tall (122 meters) in its final configuration — could deliver NASA astronauts to an asteroid and even Mars sometime in the future. [See images of NASA's SLS rocket design]
NASA's giant Space Launch System, or SLS, is derived from proven technology used for decades in America's moon program and the space shuttle. See how NASA's Space Launch System mega-rocket works in this Space.com infographic.
Credit: Karl Tate, SPACE.com
Credit: Karl Tate, SPACE.com
"Our nation is embarked on an ambitious space exploration program, and we owe it to the American taxpayers to get it right," NASA associate administrator Robert Lightfoot, said in a statement. "After rigorous review, we’re committing today to a funding level and readiness date that will keep us on track to sending humans to Mars in the 2030s – and we’re going to stand behind that commitment."
NASA expects that SLS will cost a total of $7 billion from February 2014 through November 2018. For its first test flight, SLS will fly out of low-Earth orbit with an unmanned Orion space capsule.
The SLS team just passed a major design review, which will allow the program to move forward with design plans.
The 2018 date is a reflection of modeling done by a review board, which suggested that the new date is likely more attainable, NASA officials said during a news conference today (Aug. 27). The review board looked at the SLS plan and brought up problems that could arise during the building of the rocket system, possibly causing a change in schedule.
"They're [the review board] telling us that if we don't do anything, we basically have a 70 percent chance of getting to that date," William Gerstenmaier, NASA's associate administrator for the Human Explorations and Operations Mission Directorate, said during the news conference. "Our intent and the team's intent at Marshall [Space Flight Center] is to go look at those problems and see what we can do to mitigate those problems."
"There are probably some other problems that aren't even identified by the Standing Review Board that will come up," Gerstenmaier added. "Our job as a management team is to look at those problems, figure out ways to work those ahead of time, and proactively work those as they come about."
It's possible that the first SLS flight could occur before the 2018 target if the team works to head off any potential issues before they occur, according to Gerstenmaier
¿La NASA ganó la carrera espacial?
If the space race between the United States and the Soviet Union were an athletic competition, it would look more like a decathlon than a linear 5K. Allowing each country to flex its Cold War muscles, the space race of the 1960s symbolized the power struggle between the United States and the USSR during that tenuous time after World War II.
The first successful orbit of the Soviet's artificial satellite Sputnik Ion October 4, 1957 sent the United States into a galactic frenzy. Roughly the size of a watermelon, Sputnik was the starting pistol that launched the space race. In the struggle to catch up, U.S. President Eisenhower signed into creation the National Aeronautics and Space Administration in 1958. From here, NASA and its astronauts would take center stage in the peacetime pursuit to beat the Soviet Union and its cosmonauts.
On May 25, 1961, President Kennedy announced what would become the space race's finish line: themoon. Following cosmonaut Yuri Gagarin's inaugural trip into space, Kennedy's vow to get a man on the moon by 1970 raised the bar in the space rivalry with something that would overshadow the USSR's previous string of successes. As though playing an international game of poker, Kennedy put all the American chips on the table.

In 1961, President Kennedy announced the U.S. goal to get a man on the moon. See more astronaut pictures.
Rob Atkins/Getty Images
President Kennedy's proclamation was a deft political move [source:Stenger]. In fact, both the United States and the Soviet Union spied on each other using satellite photography that was folded into their space agendas [source:National Air and Space Museum]. For instance, the U.S. satellite Corona took more than 800,000 photographs from 1960 to 1972 to learn just how much detail the images could capture [source:National Air and Space Museum].
With all this going on, who crossed the finish line first? Since the United States would put a man on the moon in 1969, did that effectively end the race? Read on to find out.
fuente: http://science.howstuffworks.com/nasa-space-race.htm?mkcpgn=fb6&utm_source=facebook.com&utm_medium=social&utm_campaign=hswaccount
Por qué Apolo 17 no despegó
On December 11, 1972, Apollo 17 touched down on the Moon. This was not only our final Moon landing, but the last time we left Low Earth Orbit. With the successful launch of the Orion capsule, NASA is finally poised to go further again. So it's important to remember how we got to the Moon — and why we stopped going.
Crewed by Commander Eugene A. Cernan, Command Module Pilot Ronald E. Evans and Lunar Module Pilot Harrison P. Schmitt, the Apollo 17 mission was the first to include a scientist. The primary scientific objectives included "geological surveying and sampling of materials and surface features in a preselected area of the Taurus-Littrow region; deploying and activating surface experiments; and conducting in-flight experiments and photographic tasks during lunar orbit and transearth coast."
Harrison 'Jack' Schmitt had earned his PhD in Geology from Harvard University in 1964, and had worked for the United States Geological Survey and at Harvard University before going through Astronaut training in 1965. Apollo 17 was his first mission into space, and would be the first astronaut-scientist to step on the surface of the Moon. Accompanying him was Eugene 'Gene' Cernan, a veteran astronaut who had first flown into space with the Gemini IX-A mission in 1966 and later served as the Lunar Module Pilot for the Apollo 10 mission in May of 1969, where he came within 90 miles of the Lunar surface.
04 14 21 43: Schmitt: Stand by. 25 feet, down at 2. Fuel's good. 20 feet. Going down at 2. 10 feet. 10 feet -04 14 21 58: Schmitt: CONTACT.04 14 22 03: Schmitt: *** op, push. Engine stop; ENGINE ARM; PROCEED; COMMAND override, OFF; MODE CONTROL, ATT HOLD; PGNS, AUTO.
Schmitt landed the Challenger Lunar Module in the Taurus-Littrow lunar valley, just to the southeast of Mare Serenitatis, a region of geological significance on the Moon. The mission's planners hoped that the region would provide a wealth of information about the history of the Moon's surface. Upon landing, the pair began their own observations of the lunar surface:
04 14 37 05: Cernan: "You know, I noticed there's even a lot of difference in earthshine and - and in the double umbra. You get in earthshine on the thing, and it's - it's hard to see the stars even if you don't have the Earth in there."04 14 23 28: Cernan: "Oh, man. Look at that rock out there."Schmitt: "Absolutely incredible. Absolutely incredible."
After several hours of preparation, Cernan stepped onto the Lunar surface:
04 18 31 0: "I'm on the footpad. And, Houston, as I step off at the surface at Taurus-Littrow, I'd like to dedicate the first step of Apollo 17 to all those who made it possible. Jack, I'm out here. Oh, my golly. Unbelievable. Unbelievable, but is it bright in the Sun. Okay. We landed in a very shallow depression. That's why we've got a slight pitch-up angle. Very shallow, dinner-plate-like."
The two astronauts unloaded a lunar rover, and began to deploy scientific instruments around their landing site: an experiments package and explosives (to complete seismic experiments begun with other Apollo missions in other locations on the Moon). Their first exclusion in the rover yielded numerous samples of lunar rock. Over the next couple of days, the astronauts completed two additional Moon walks, where they continued to drive across the lunar surface and collect samples.
Schmitt later described the landing site to NASA Oral historian Carol Butler: "It was the most highly varied site of any of the Apollo sites. It was specifically picked to be that. We had three-dimensions to look at with the mountains, to sample. You had the Mare basalts in the floor and the highlands in the mountain walls. We also had this apparent young volcanic material that had been seen on the photographs and wasn't immediate obvious, but ultimately we found in the form of the orange soil at Shorty crater."
Why we went to space
The scientific endeavors of Apollo 17 were the culmination of a massive program that had begun in 1963 following the successes of the Mercury Program. In the aftermath of the Second World War, the United States and Soviet Union became embroiled in a competitive arms race that saw significant military gains on both sides, eventually culminating in the development of rockets capable of striking enemy territory across the world. The next step for arms superiority jumped from the atmosphere to Low Earth Orbit to the Moon, the ultimate high ground. As this happened, each country capitalized on the advances in rocket technology to experiment with human spaceflight missions. The Soviet Union succeeded in putting Yuri Gagarin into space in 1961, just a couple of years after launching the first satellite into orbit.
Closely followed by the United States, space became an incredibly public demonstration of military and technological might. The development of space travel didn't occur in a political vacuum: the drive for the United States to develop rockets and vehicles which could travel higher and faster than their Soviet counterparts happened alongside increasing US/USSR tensions, especially as geopolitical crises such the Cuban Missile Crisis and the US deployment of missiles to Turkey demonstrated how ready each country was to annihilating the other.
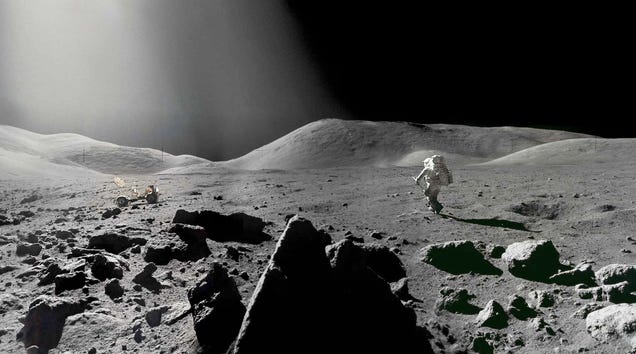
Image via Universe Today
As the space program took off, it was supported by other research and scientific efforts from the broader military industrial complex which President Dwight Eisenhower had worried about just a handful of years earlier. (Eisenhower had not been a major supporter of the development of space travel which began under his watch, and had attempted to downplay the significance of Sputnik.) The red hot environment of the Cold War allowed for significant political capital and governmental spending which supported a first-strike infrastructure, and in part, trickled over to the scientific and aeronautical fields, which maintained a peaceful and optimistic message.
By 1966, the space race peaked: NASA received its highest budget ever, at just under 4.5% of the total US federal budget, at $5.933 billion dollars (around $43 billion today.) The United States had made clear gains in space by this point: Project Gemini had completed its final mission, and with efforts towards the next phase under Apollo were well under way. By this point, the social and political infrastructure and support for space had begun to wane, and would ultimately fall away after Apollo 11 successfully landed on the Moon's surface in July of 1969. After this point, NASA continued with planned missions, and eventually landed five additional Apollo missions on the Moon. (Another, Apollo 13, was unable to land after mechanical problems).
Changing priorities
Just a year after Apollo 11 landed, NASA began to reprioritize: plans for a space station were revived, and in 1970, they announced that Apollo 20 would be cancelled in favor of the creation of a new venture: Skylab. On September 2nd, 1970, the agency announced the final three Apollo missions: Apollo 15, 16 and 17. The agency was forced to contend with political pressure as well: In 1971, the White House intended to completely cancel the Apollo program after Apollo 15, but ultimately, the two remaining Apollo missions were kept in place. Harrison Schmitt, who had been training for Apollo 18, was bumped up to Apollo 17 after NASA faced pressure from scientists to send one of their own to the Moon.
On December 14th, 1972, Cernan became the last human to step on the Moon's surface:
07 00 00 47: "Bob, this is Gene, and I'm on the surface and as I take man's last steps from the surface, back home, for some time to come, but we believe not too long into the future. I'd like to Just list what I believe history will record that America's challenge of today has forged man's destiny of tomorrow. And, as we leave the Moon at Taurus Littrow, we leave as we come and, God willing, as we shall return, with peace and hope for all mankind. Godspeed the crew of Apollo 17."
In the forty-two years since those words were spoken, nobody has stepped on the Moon. The levels of federal spending which NASA had received before 1966 had become untenable to a public which had become financially wary, particularly as they experienced a major oil crisis in 1973, which shifted the nation's priorities. Spending in space was something that could be done, but with far more fiscal constraints than ever before, limiting NASA to research and scientific missions in the coming years. Such programs included the development of the Skylab program in 1973, and the Space Shuttle program, as well as a number of robotic probes and satellites.
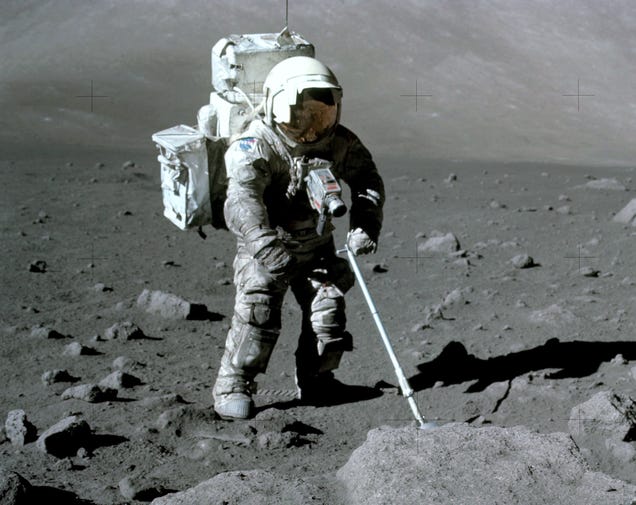
This shift in priorities deeply impacted the willpower of policymakers to implement new exploratory missions to the Moon and beyond. Optimistic dreams of reaching Mars had long since perished, and as NASA focused on the Space Shuttle, the physical infrastructure which supported lunar missions vanished: No longer were Saturn V rockets manufactured, and unused rockets were turned into museum displays. The entire technical and manufacturing apparatus, which has supported both military and civilian operations, had likewise begun to wind down. The Strategic Arms Limitation Talks (SALT) and its successors began to freeze the numbers of missiles which could be deployed by both the United States and Soviet Union in 1972, and each country largely began to step down their operations. The urgency which fueled the Cold War arms race had begun to cool, and along with it, the support for much of the efforts required to bring people into space and to the Moon.
Since that time, US Presidents have spoken of their desire to return to the Moon, but often in terms of decades, rather than in single digits. It's easy to see why: up until recently, US spaceflight operations were focused entirely on Low Earth Orbit activities, as well as admirable cooperative international programs such as the International Space Station, and major scientific instruments such as Mars Pathfinder, Opportunity/Spirit and Curiosity. Other major concerns have redirected US attentions from spaceflight: the United States' War on Terror, which is expected to cost US taxpayers over $5 trillion dollars in the long run.
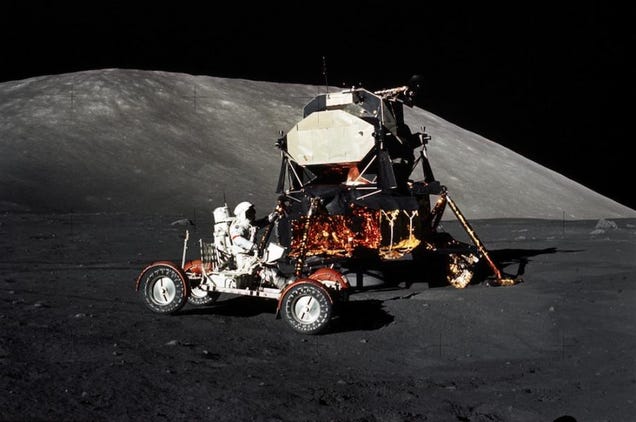
The launch of Orion atop a Delta IV Heavy rocket was exciting to watch, as well as newer players in the space launch field, SpaceX and Orbital Sciences Corporation, which suggesting that a new generation of infrastructure is being constructed. The reasons for visiting the Moon and potentially, other planets and bodies in our solar system, are numerous: they could be the greatest scientific endeavors of our existence, allowing us to further understand the creation of our planet and solar system and the greater world around us. More importantly though, such missions contribute to the character of the nation, demonstrating the importance of science and technology to our civilization, which will ultimately help us process and address the issues of greatest concern: the health of our planet. Hopefully, Cernan's words and hope that our absence from the Moon will be short-lived, and that we will once again explore new worlds in our lifetimes.
Suscribirse a:
Entradas (Atom)